1. Introduction
As a result of the population increase over the past few decades, the increase in the consumption of electric energy is directly proportional to the consumption of fossil fuels. The main cause of environmental pollution, climate change, and global warming is a result of carbon emissions from fuels (1). Statistical studies showed that countries with hot climates, in particular, are the most consuming of electricity and fossil fuels in air conditioning see for to provide suitable comfort conditions (2). Recently, many researchers have made several attempts to save part of the energy consumed by using hybrid cooling systems by exploiting renewable energy sources accompanying the environment as some developments have been made to the traditional air-conditioning system by adding thermal solar collectors, which depend on the amount of incident solar radiation to reduce the amounts of energy that are expenditure during the cooling cycle (3). Bramankar et al.(4) presented a study on the performance of the system in India’s climatic conditions. The results of the research summarized that the electrical energy saving rate amounted to 29−30% of the total energy consumed after comparing the two hybrid and conventional units, and thus, helped reduce the amount of carbon emitted from energy and its generation. Rahman et al. (5) stated that optimization of a solar hybrid cooling system was conducted by adding a 5% secondary single-wall tube size in the cooler. The temperature range was recorded between (283 and 308) in addition to reducing the cooling load by misleading the building, reflective walls, and ceilings, reducing the size of the windows, and adding double glazing to the windows, which led to a reduction of 31.5% of the total cooling load. The results of the material’s thermal conductivity were cooling (R-407c) at 305k with an increase in a pressure rate of 4%. SWCNT/R-407c nano refrigerant shows a notable improvement in coefficient of performance (COP) at about 17.02% for thermal conductivity and at about 10.06% for specific heat, which saved 34% of the total electrical energy consumed in the system, and increased COP by 4.39% compared with the traditional adaptation regime. Providing cooling loads is during the hot summer in Iraq, as it is among the most important countries that enjoy a high abundance of sustainable energy. In the literature, several researchers have studied the feasibility of using clean solar energy to provide a cooling load and reduce the consumption of hybrid air-conditioning systems. Vakiloroaya et al.(6) presented a project aiming to reduce the amount consumed for savings at a rate of 25−45% per month, with a cooling capacity of 6°kW during climatic conditions in the city of Baghdad. He worked on developing the performance of the hybrid air-conditioning system, by adding an inline solenoid valve after the compressor that controls the amount of gas flowing to the solar heater. Assadi et al.(7) presented a theoretical analysis of a hybrid air-conditioning system using ANSYS-FLUENT simulation software. The amount of energy saved was 45% of the total energy. A comparison between the cooling systems with a cooling capacity of 3.5°KW and with different dimensions for evacuated tube solar collectors was studied during this research with the conventional cooling system. Kaidir et al.(8) used a 3, 51°KW, (12,000 Btu/h) hybrid air-conditioning system. Refrigerant type R22, in the climatic conditions of the city of Indonesia. A hot water tank with a capacity of 22.5 liters to 120 liters was used. In the first tank, the temperature was raised at a rate ranging from 50 to 65°C, which has a capacity of 22.5 liters within 105 min, while the temperature of the second tank with a capacity of 120 liters was raised from 27 to 62°C within 240 min. A saving rate was obtained. The energy rate is 60% of the total electrical energy, and the performance factor for the three hybrid systems with different capacities and the traditional tanks is COP = (3.35, 8.86, and 9.85) for the A, R, and C systems, respectively. The operating rate of the system was 6 h per day for the maximum temperature of the hot water that was recorded at 88.5°C. Anoop Kumar and Patel (9) presented an analytical comparison of the research findings of a hybrid air-conditioning system with a conventional refrigeration system. The results showed that the energy saving rate ranged from 25 to 40%. The selected system has an average energy saving of 1.3% in the on-off mode of operating the compressor, while if the compressor is in continuous operation, there is no energy saving. Through this process, 30% of the total energy can be saved. Kinnal et al.(10) discussed a special type of solar system designed in a simplified manner and with available materials that can be designed by researchers at home without the need for expertise and complexity. The water tank was designed with a glass front to allow the penetration and absorption of solar radiation, and the inside of the tank was painted with a dark material or paint to absorb as much solar energy as possible. The work of the hot water tank was to increase the temperature and pressure of the refrigerant leaving the evaporator and going to the compressor in a hybrid cooling system (a conventional air conditioner connected to a solar collector). This study was conducted during the months of April and May for a cooling system with a capacity of 3.5 kilowatts, and during the peak summer, temperatures were recorded from 1:30 p.m. to 4:00 p.m. during the period from 3:00 to 3:30 p.m. at a temperature of 51°C. Energy savings averaged 32% over the 3°months, while it averaged 45% during the peak summer season. System performance has been improved from 2.85 to 4.04 = COP. During this whole process and after comparing the two systems, it was found that it is possible to reduce the electric power consumption of the compressor by 30−40%. This hybrid air-conditioning system is cost-effective and environmentally friendly.
This study aimed to study and design a hybrid solar absorption air-conditioning system for the climatic conditions of Thi-Qar, Iraq. It differs from existing research as it involves the study of building parameters and their configuration to provide thermal comfort in hot environments and uses R-410A as the heat transfer fluid. In addition, the analysis and parameter quantification are performed for thermal reasons, and the results are presented in the form of temperature and energy gain.
2. System description
A hybrid solar air-conditioning system is shown in Figures 1A, B, and the working basis of the system consists of a condenser, compressor, evaporator, pressure valve, and helical coil heat exchanger with a vacuum tube solar heater collector. In this paper, two cases have been suggested. In the first case, the heat exchanger is connected between the compressor and the evaporator. It is assumed that during this process, first, the compressor voltage is reduced, and second, which is accompanied by a decrease in electricity consumption. The cooled gas is passed through the spiral heat exchanger, which is installed inside the hot water tank coming from the solar collector, increasing the temperature of the refrigerant, and thus, leading to an increase in vapor pressure according to the ideal gas equation PV = M.R.T. To heat a constant volume as shown in the process 1-x-2-3-4.

Figure 1. (A) The Hybrid solar air conditioning system in case one. (B) Pressure-Enthalpy Diagram for case one.
While in the second case, a heat exchanger was installed between the compressor and the condenser as shown in Figure 2A. Thus, the purpose of this process is to try to improve the performance of the cooling system and claim that it reduces electricity consumption by reducing the operating time of the compressor (compressor off). The hot water coming from the solar collector is drained into the tank to raise the temperature of refrigeration through the solenoid, which aims to conduct the heat exchange process between the hot water and the pressurized refrigerate from the compressor toward the condenser. Thus, the refrigerant is heated at a constant volume according to the ideal gas equation of state and as shown in the process line (1-2-x-3-4) in Figures 2A, B:

Figure 2. (A) The Hybrid solar air conditioning system in case two. (B) Pressure-Enthalpy Diagram for case two.
During this process, the pressure is assumed to increase with the temperature increase. So it is suggested the possibility of continuing the work of the refrigeration cycle even when the compressor is idle in the off phase using the pressure provided by the vacuum solar collector, and finally, the two claims must be validated by an empirical analysis of both the attempts made in this research.
3. Mathematical
3.1. Thermal analysis of conventional air-conditioning system (CVCRC)
A thermodynamic analysis of the system was conducted to try to reach the possibility of energy savings and to determine the impact of different operating conditions on its performance. By applying the energy balance equation to the system and its various components, we can arrive at the following relationships (9):
Cooling capacity of the evaporator (qevap)
Refrigerant mass flow rate through the compressor:
Compressor work (Wcomp)
Coefficient performance of the system, (COPc)
Heating capacity at the condenser, (qcond)
where:
h1: enthalpy at the inlet of compressor, respectively, in (KJ/Kg).
h2: enthalpy at the exit of compressor, respectively, in (KJ/Kg).
h3: is the enthalpy at the exit of the condenser in (KJ/Kg).
h4: is the enthalpy at the inlet of the evaporator in (KJ/Kg).
hout: the enthalpy at the exit of water or collector in (KJ/Kg).
hin: the enthalpy at the inlet of water or collector in (KJ/Kg).
3.2. Analysis of modified air-conditioning system
3.2.1. Case 1
For a standard system, an attempt is made to heat up the refrigerant before entering the compressor and the exit from the evaporator, which operates in the first state points 1-x-2-3-4. From state 1 to state x, it is compressed to state 2 as shown in Figures 1A, B. The values of the specific cooling effect can be calculated by the following equations (11):
Cooling capacity of the evaporator (qevap)
Refrigerant mass flow rate through the compressor:
Compressor work (Wcomp)
Coefficient of performance of the modified system, (COPc)
Heating capacity at the condenser, (qcond)
Energy saving (ES) represents the ratio of saving in work due to adding solar that comes to the total work (7):
Heating capacity at the collector, (qcoll)
To calculate the average pressure at point x through the following relationship:
To calculate the heating of the refrigerant mass in the heat exchanger:
where Mhx is mass in the heat exchanger, mw is the mass flow rate of water in the collector, hW out and are the entropies of water exit and inlet to the water heater, Vhx is the volume of the coil of the heat exchanger (0.01185 m3), and Vx is the specific heat of the refrigerant vapor in the x state. The performance factor of the hybrid system is calculated based on the amount of electrical energy consumed in the compressor (9), while the heat generated by solar radiation is free and is not considered in terms of energy input (free energy):
where hx is the enthalpy at state x.
3.2.2. Case 2
In this case, the solar collector is added after the compressor (between compressor and condenser) for the second case points 1-2-x-3-4, as shown in Figures 2A, B. The refrigerant is transferred from state 2 to state x and from state x to state 3, the process is constant volume heating in the heat exchanger for the second case, standard vapor pressure system operating at processing points such as 1-2-x-3-4, and the specific cooling effect is according to the cooling capacity, which was calculated by the following equation (9):
Cooling capacity of the evaporator (qevap)
Refrigerant mass flow rate through the evaporator:
Compressor work input (Wcomp)
Coefficient of performance of the modified system (Condenser heating capacity, (qcond)
Energy saving (ES)
Calculated solar collector heating capacity or cycle additive work (free heat energy added from the solar collector) and (qcoll)or (Ws) radiation energy comes from thermal solar collector tubes.
Heating capacity at collector or work saved compassed (qcoll):
3.3. Thermal analysis of solar thermal collector
As mentioned earlier, the main purpose of adding solar collectors to conventional refrigeration systems at these two locations is to increase the temperature and pressure of the refrigerant in an attempt to increase the performance of the system and reduce energy consumption. Solar heaters increase water temperature by absorbing the sun’s rays falling on hollow glass tubes. The tubes are evacuated from the air to reduce the process of energy loss and heat transfer to the outside to improve the insulation process. They provide maximum heat from solar radiation reflected by heaters.
3.3.1. Thermal analysis of evacuated tube
To obtain the appropriate thermal quantities to conduct the process of heating the water to the required temperatures to complete the heat exchange between the refrigerant inside the solenoid coil and the hot water inside the tank well, an analytical study was conducted for the required dimensions of the solar collector as shown in Figures 3, 4, and the required heat capacity through the mathematical relationships is shown below (12).
To calculate the required heat capacity:
The rate of heat loss per unit absorbed area qLoss can be expressed as follows:
or
where Tr is the receiver temperature, Ta is the ambient air temperature, Tp is the absorber plate temperature, Tout is the outlet temperature of water or refrigerant, Tin is the inlet temperature of water or refrigerant, UL is the loss coefficient, and Ap is the loss area.
Total thermal resistance 1/UL is the sum of three resistances:
R1 = radiative exchange from absorber tube to cover tube
R2 = conduction through the glass tube
R3 = convection and radiation to the environment
Then, the overall resistance is as follows:
It was found that the recorded loss coefficient UL varies between (0.5 and 1.0) W/m2 C, according to experimentally proven data (12).
The actual total heat capacity that can be obtained from the solar collector after the process of subtracting the heat capacity recorded losses:
or it can be written as the mathematical equation in the following simplified form, through the process of abbreviation that took place from adding the area law to the main equation and calculated from the following law (A = πDr/d) to get the mathematical formula for calculating the total heat capacity of the solar collector
where Dt is the outlet diameter tube (58 mm), d is the center diameter tube (47 mm), τe is the transmittance of the collector caver (between 0.6 to 1%), αr is the solar absorptance of the collector (between 0.96 and 0.91), Ieff is the effective solar radiation, At is the projected area, and Ac is the cross sectional area (12, 13).
3.3.2. Thermal analysis of heat exchanger
Their heat balance for the heater is shown in Figure 5, as follows (13):
The heat transferred to the refrigerant can be calculated from the following equation:
The heat loss transferred from the water can be calculated from the following equation:
4. Results and discussion
This section describes the effectiveness of the proposed approach on system performance. The test facility contains two cases; in the first case, the solar collector is inserted before the compressor, while it was connected after the compressor in the second case to heat the refrigerant coming from the compressor.
The hybrid solar air-conditioning system proved highly efficient in saving energy and improving practical cooling performance. An analytical study was conducted for a hybrid system with a cooling capacity of 1 ton (3.51 kW) using refrigerant of type R-410a, which is environmentally friendly; in the climate of Nasiriyah city, south of Iraq during the summer season, where the rate of solar radiation reaches 1,000 W/m^2 and according to numerical analysis using the approved arithmetic rates to calculate all the necessary data.
The analytical study was conducted for the previous two cases on hot climatic conditions in Nasiriyah city, south of Iraq located on longitude (46.14O) and latitude (31.15O), which has a direct impact on the angle of incidence of solar rays. Additionally, a solar radiation rate of up to 1,000 W/m^2 was measured using a radiometer.
Figures 6A, B show the variation of the energy savings with the collector temperature for two cases (the first and the second cases). From these figures, it can be seen that the energy savings increase with the increase in water temperature of the collector in both cases. Due to the increase in the temperature and pressure of the refrigerant, the pressure is directly proportional to the temperature, which reduces the amount of energy required to compress and circulate the refrigerant inside the pipes. In the first case, the energy saving rate is up to 67% if the rotary compressor used is able to withstand high return refrigerant temperatures of more than 70°C, while the optimum refrigerant temperatures at which the compressors can operate do not exceed 55°C. Therefore, the highest rate that can be saved from the available refrigeration compressors does not exceed 51% of the amount of electrical energy consumed within the return refrigeration temperatures of up to 55°C. While the results of the second case indicated, the rate of energy saving reaches 24% when the highest average temperature of 160°C is obtained from the available solar collector with a solar collecting area (1.8 m2). From this figure, it can also be seen that the energy saving of the first case is higher than that of the second because the temperature obtained from the collector for the second case is higher than the temperature collected from the collector for the first case.

Figure 6. Variation of energy savings with the collector temperature for two cases (case one and case two). (A) Case one. (B) Case two.
Figures 7A, B show the variation of COP with the collector temperature for two cases (case one and case two). From this figure, the COP starts from 3.37 in the original case. The original values were selected from the sources mentioned and relied upon in the calculations of this paper. The rate increases from 3.85 to 51 with an increase in the water temperature of the collector in the first case. From this figure, it can be seen that COP increased in the second case by an average of 5.1 to 13. The main reason for the increase in the performance factor in the first case that is higher than in the second is the reduction in the amount of energy consumed in the compressor cycle and as a result of an increase in the temperature of the refrigerant going to the compressor at a temperature from 30 to 70°C, where the movement of particles is directly proportional to the temperature of the refrigerant inside the pressurized tubes allowing the gas to be ejected and compressed toward the condenser and thus, accompanied by an improvement in the amount of drain and a reduction in the working effort of the spent compressor to push the gas into the condenser very significantly, while in the second case, the gas is heated after the compressor, which helps to reduce only 20% of the consumed compressor effort and increase the amount of cooling from 3.5 to 5.5, which is one of the advantages of the second case, which is more beneficial than the first. From this figure, it can also be seen that the COP in the second case is less than in the first because the amount of energy expended in the first case is much less.
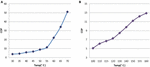
Figure 7. Variation of the temperature refrigerant supplied from the solar collector tube with the coefficient of disease COP in two cases. (A) Case one. (B) Case two.
Figures 8A, B show the variation in cooling capacity using a temperature collector for two cases (cases one and two). From this figure, it can be seen that the average cooling capacity is constant in the first case with a capacity of 3.43 kW, while it increases with the increasing water temperature of the collector in the second case from 3.5 to 5.5 kW. The main reason is due to the increase in the amount of coolant temperature and pressure, where the pressure is directly proportional to the recorded temperature from 100 to 160°C, and as a result of the improvement in the saturation temperature and approaching the tipping point on the saturation line of the refrigerant and resulting from the increase in pressure and accompanied by an increase in the rate flow inside the pressure valve. Thus, it allows for a faster phase change and lower coolant temperature. As a result, the improvement in enthalpy helps in increasing the cooling and heat exchange process, and it can be seen that the cooling rate is stable in the first case as a result of not changing the temperature and pressure of the refrigerant leaving the compressor and going to the condenser and then going to the pressure valve stage as in its original state without any improvement in coolant conditions. It can also be noted that the cooling rate stops when the refrigerant reaches a temperature of more than 70°C as a result of the cycle compressor stopping working (reaching the shutdown) as a result of exceeding the practical conditions of the compressor. From this figure, it can also be seen that the amount of cooling in the first case is less than that of the second case, and the result is a change in the coolant conditions because the temperature obtained from the collector of the second case is higher than the temperature collected from the collector of the first case, resulting in the process that improves the tangible temperature of the coolant (enthalpy).

Figure 8. Variation of the cooling capacity of the evaporator with collector temperature for two cases (case one and case two). (A) Case one. (B) Case two.
Figures 9A, B show the contrast of the heat removed in the condenser with the collector temperature for two cases (cases one and two). From this figure, it can be seen that in the first case, the capacitor capacitance rate is constant at a rate of 4.2 kW, while the second indicates an increase with an increase in the water temperature of the collector from 100 to 160°C, at a rate from 4.2 to 6.7 kW. Due to the increase in the amount of heat added from the solar collector to the refrigerant, it can be seen that the heating rate is stable in the first case due to the unchanged temperature of the refrigerant transferred to the condenser. The conditions experienced by the refrigerant in the first case are very similar to what they are in the conditions of the original case of the adaptive system during the condenser phase. The reason is that the solar heater connected before the compressor is only limited to helping the compressor circulate the gas without improving the enthalpy (↑Δh), as the gas exit from the compressor is similar to the conditions of the original case, and the reason was previously mentioned for this case within the available heating limits that can be added to the gas before entering the gas compressor. From this figure, it can also be seen that the heating capacity of the first case is constant and much less than that of the second case because the amount of heat obtained and added to the refrigerant at a rate of 100–160 °C from the collector of the second case is higher than the combined temperature of the first case from 30 to 70 Celsius.
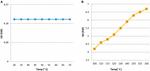
Figure 9. Variation of the rate heating capacity for the condenser with the collector temperature for two cases (case one and case two). (A): Case one. (B): Case two.
Figure 10 shows the variance in COP system amplitude and system strength with work required for two cases studied (case one and case two). From there it can be seen that the rate of the refrigerant capacity is constant in the first case with an increase in the rate of the performance factor due to the reduction of the amount of electrical energy consumed in the compressor. In turn, the second case indicates an increase with decreasing work as the decrease in compressor work was compensated for by an increase in the amount of heat added from the accumulator to the refrigerant. This results in an improvement in the physical conditions of the coolant such as the saturation temperature, the approaching inflection point on the saturation line, increased particle movement, and the speed of the cooling phase change. This is an increase in the flow velocity within the pressure valve stage, which allows for greater cooling of the coolant temperature and an increase in the heat exchange rate load, thus improving the enthalpy of the gas. It can be seen that the cooling rate is stable, and the performance factor increases with a decrease in the compressor’s work in the first case where the gas is heated directly before the compressor to reduce the amount of energy consumed without going through the overheating stage before entering the condenser stage only. It can be seen that the properties of the refrigerant are similar to the original conditions in a conventional air-conditioning system. From there, it can also be seen that the refrigerant capacity of the first case is much lower than that of the second because the temperature and pressure obtained and added to improve the refrigerant properties of the second case collector helped reduce the amount of workload, reducing heat and energy expenditure in the compressor above the combined temperature of the first case, which was limited only to balance the amount of energy the compressor provides to circulate the refrigerant.

Figure 10. Variations of coefficient of performance (COP) and system cooling capacity QL with required work for two cases studied.
5. Conclusion
The results discussed for the performance of hybrid air-conditioning systems with solar energy in the weather of the city of Nasiriyah have been made in two cases.
-Case one was the solar heater that was connected before the compressor
- Case two was the heater that was connected after the compressor.
From the obtained rustle, the following conclusions were made:
Renewable energy has been utilized. The heat emitted by solar radiation ranges from 0 to 1000 W/m2 and averages 947 W/m2 per day.
The solar heater was connected before the compressor in the first case, and the gas was heated from 30 to 70°C through the heater. The recorded results showed increased energy savings with the increase in heater temperatures with an average of between 25 and 55% of the total energy consumed in the refrigeration cycle per day. The average work of the compressor ranges between 0.8904 and 0.016 where the gas is heated to temperatures ranging from 45 to 60°C and the highest temperature that the compressors can tolerate.
The solar heater was connected after the compressor in the second case. The solar heater provided a significant improvement in the system’s performance coefficient with an average range of 5.1–13 and an amount of energy ranging from 12 to 24% of the total energy consumed. Thus, the improvement of the cooling load can be observed from 3.43 to 5.5 kW with temperatures from 100 to 160°C gradually.
Through the results, it can be seen that the second case has a service advantage and much greater economic feasibility than the first case.
Solar energy in terms of consumption of electric energy, economic cost, and its impact on the environment is more effective than commercial refrigeration systems.
This proposed solar system is incompatible, economical, and efficient with the weather in Iraq.
6. Nomenclature
qevap: Cooling capacity of the evaporator [KW]
V: Volume [m3]
R: Ideal gas constant [kJ/kg.K]
Cop: Coefficient of performance [-]
Cp(R–410A): Heat capacity R-410a at constant pressure (0.23901)[kJ/kg.K]
Cp(Water): Heat capacity water at constant pressure (4.19)[kJ/kg.K]
C1 and C2: cases one and two.
△h: Insercece change enthalpy
m: Mass of heated refrigerant [Kg]
hx : Enthalpy at state x
Wcomp: Compressor work [Kw]
COPc : Coefficient of performance of modified system [-]
qcond: Heating capacity at condenser [KW]
qcoll : Heating capacity at collector [KW]
qLoss : Heat loss per unit absorbed area [KW]
HSAC: Hybrid solar air conditioner
P: Absolute pressure [kPa]
Author contributions
MS: A review of the study of the solar hybrid air-conditioning system, Renewable, and Sustainable Energy Reviews. Investigation of the solar-powered hybrid air-conditioning system with different approaches: A review. MH: Fabricating and testing of the ground coupled air conditioner for residential applications in Iraqi weather. Both authors contributed to the article and approved the submitted version.
Acknowledgments
We would like to thank my supervisor (MH) for helping me with this project. He allowed me to work on this project.
Conflict of interest
This research was conducted in the absence of any commercial or financial relationships that could be interpreted as a potential conflict of interest.
References
1. Urge-Vorsatz D, Cabeza LF, Serrano S, Barreneche C, Petrichenko K. Heating and cooling energy trends and drivers in buildings. Renew Sustain Energy Rev. (2015) 41:85–98.
2. Sevinç K, Güngör A. Güne enerjisi kaynakli soğutma sistemleri ve bu alandaki yeni uygulamalar. Mühendis Makina. (2012) 53:59–70.
3. Fong K, Lee C. Investigation on effect of indoor air distribution strategy on solar air-conditioning systems. Renew Energy. (2018). doi: 10.1016/j.renene.2018.07.065
4. Brahmankar C, Hrishikesh B, Akshay G, Himanshu R. Study of solar-thermal collector assisted hybrid split air conditioner. Int Res J Eng Technol. (2018) 5:2921–5.
5. Rahman S, Issa S, Said Z, El Haj Assad M, Zadeh R, Barani Y. Performance enhancement of a solar powered air conditioning system using passive techniques and SWCNT /R-407c nano refrigerant. Case Stud Therm Eng. (2019) 16:100565.
6. Vakiloroaya V, Ismail R, Ha QP. Development of a New Energy-Efficient Hybrid Solar-Assisted Air Conditioning System. Sydney: School of Electrical, Mechanical and Mechatronic Systems, University of Technology (2013).
7. Assadi K, Gilani SI, Jun Yen TC. Design a solar hybrid air conditioning compressor system. MATEC Web Conf. (2016) 38:02001.
8. Kaidir, Mulyanef, Burmawi. Performance study on a solar hybrid air-conditioning system for residential water heating. Int J Civil Eng Technol. (2017) 8:706–14.
9. Anoop Kumar M, Patel D. Performance assessment and thermodynamic analysis of a hybrid solar air conditioning system. Mater Today Proc. (2020) 46:5632–8.
10. Kinnal N, Ashok Kumar MS, Muthukumaran J, Santhosh DN, Shivaprakash KS. Hybrid air-conditioning system using solar heater. J Therm Energy Syst. (2020) 5.
11. Hasan MI, Jabbar EK. Fabricating and testing of the ground coupled air conditioner for residential applications in Iraqi weather. Energy. (2021) 216:119256.
12. Goswami D. Chapter 3, Solar thermal collectors. 3 ed. Principles of Solar Engineering. Boca Raton, FL: CRC Press (2015). p. 119–58.