1. Introduction
Cr-Mo are the materials used in tube/pipe components in various industries owing to their creep strength, oxidation, and corrosion resistance (1–4). The steels are also used with their modifications by suitable addition of carbide-forming elements such as Nb, V, Ti, or W (5–7). Microstructure of the steels consisted of ferrite-pearlite, bainite, or tempered martensite mostly used in annealed, normalized tempered, or quenched tempered conditions (8). However, long-term exposure of the steels under aggressive environments such as high temperature in the range of 350°–600°C, stress in the range of 15–30 MPa, and corrosive environments lead to deterioration of microstructure and mechanical properties (1, 3, 8). With excessive deterioration of the properties, catastrophic failures occurs, leading to material loss, machine downtime, and in some case manpower loss. Although the design life of the components is fixed at the time of installation, lots of components are running beyond their design life without failure, indicating that life extension of the components is possible if the health of the components is found to be good and premature failure can be prevented if the health of the components is found to be in critical condition. Hence, monitoring the components through non-destructive evaluation (NDE) is of prime importance.
Conventional NDE techniques are used for the evaluation of cracks/defects at the latter stage, while there is a need to evaluate the microstructural modifications much earlier than the void/crack generation in service. The development of suitable NDE techniques for monitoring the health of the components for precise evaluation of microstructure and mechanical properties is highly desirable. Magnetic-based NDE techniques are in growing demand for the common pinning sites for the magnetic domain wall motion and dislocation motion in steels (9–18). However, the sensitivity of the different magnetic parameters to various micro-constituents makes the technique complex to evaluate. Hence, there is a need to get more publications on the subject to better understand the complex change in properties and make the evaluation simpler. Several studies have been reported on the evaluation of Cr-Mo steels through magnetic NDE, however, no report is found on the martensitic 2.25Cr-1Mo steel degradation under service or accelerated ageing conditions. In addition, weld structures of the steels constitute various microstructures, and degradation in weld structures is much faster than that in the base metal. Therefore, health monitoring of such structures can give better safety to the steel components.
In the present study, magnetic hysteresis loop (MHL) measurement was employed to monitor accelerated-aged martensitic 2.25Cr-1Mo steel and compared with its bainitic structure during the time of interruption. Microstructure and microhardness have been measured and correlated with the magnetic parameters for the non-destructive health monitoring of the steel components.
2. Research elaborations
Chemical composition of the 2.25Cr-1Mo steel investigated is shown in Table 1. Dimension of the steel used in the experiments is 100 mm × 25 mm × 6 mm. The JMatPro software was used to construct the advanced CCT and TTT diagrams, as shown in Figures 1A, B, respectively, to obtain the transition temperatures of the steel. The transition temperatures obtained from the advanced CCT diagram were Ac1 and Ac3 as 733°C and 889°C, respectively. The bainite start temperature (Bs) is 629°C, and the martensite start temperature (Ms) is 439°C. The samples were homogenized at 1000°C/1 h, followed by water quenching and air quenching for martensitic and bainitic microstructure and subjected to accelerated thermal ageing at 700°C for various lengths of time (1, 3, 5, 25, 50, 75, 100, 300, 500, 700, and 1000 h) up to 1000 h. Microstructure and hardness were measured from a small piece of sample cut after the magnetic measurement at the time of the interruption. Optical microscopy and scanning electron microscopy (SEM) were used for the microstructural evaluation after polishing the samples to a mirror finish and etching with Nital. Microhardness measurements were carried out using Vicker’s hardness tester at 0.5 kgf. Energy dispersive x-ray (EDX) analysis was carried out for the evaluation of chemical composition of the carbides at various stages of ageing. MHL measurements were conducted on both surfaces of the steels using a portable magnetic NDE tester; MagStar with the applied field and frequency was the same as reported earlier (18). The portable equipment can be taken to the site, and using surface probes, the microstructural modifications can be monitored to assess the health of the components. As the scale on the surface of the tube materials would affect the magnetic properties, it is suggested to remove the scale before conducting magnetic measurements. As high temperature largely affects the magnetic properties, magnetic measurements should be made at room temperature only.

Figure 1. (A) Advanced CCT diagram and (B) TTT diagram of the steel obtained through JMatPro Software.
3. Results and findings
3.1. Microstructure
3.1.1. Martensitic 2.25Cr-1Mo steel
Microstructure of the steel at water-quenched condition is shown in Figures 2A–H aged at 700°C for 1, 3, 5, 25, 50, 75, and 100 h, respectively. SEM micrograph at water-quenched condition is shown in Figures 3A–D aged at 700°C for 1, 3, and 5 h, respectively, at low magnification (1000X), and (e)–(h) are their corresponding high-magnification micrographs (5000X). SEM micrograph of the steel aged at 700°C for 25, 50, 75, and 100 h is shown in Figures 4A–D at low magnification (1000X), and (e)–(h) are their corresponding high-magnification micrographs (5000X). The as-received microstructure revealed a fully lath martensitic structure with a very fine martensitic lath in the range of 0.1–0.3 μm. A large density of dislocations and compressive residual stress is expected at such conditions due to the metastable microstructure and the rapid cooling (8). With the increase in accelerated thermal ageing time, the martensitic lath broadening have been found with 10–15-folds at 100 h of ageing. A decrease in dislocation density along with the lath broadening of martensite was reported earlier for creep conditions (8).

Figure 2. Microstructure of the martensitic 2.25Cr-lMo steel at water quenched condition (A), with (B–H) aged at 70 C for 1, 3, and 5, 25, 50, 75, and 100 h, respectively, at 500X.

Figure 3. SEM Micrograph of the martensitic 2.25CrlMo steel at water quenched condition (A), with (B-D) aged at 700 C for 1, 3, and 5 h, respectively, at 1000X, (E–H) are their corresponding micrographs at 5000X.

Figure 4. Micrograph of the martensitic 2.25CrlNfo steel (A–D) aged at 700 C for 25, 50, 75, and 100 h, respectively, at 1000X, (E–H) are their corresponding micrographs at 5000X.
Microstructure of the steel aged at 700°C for 300, 500, 700, and 1000 h at 500X is shown in Figures 5A–D. The corresponding SEM micrograph of the steel aged for different lengths of time from 300 to 1000 h is shown in Figures 6A–D at 1000X with their high-magnification micrograph at 5000X in Figures 6E–H. The result shows that the carbides become massive forms with increasing age. The EDX analysis of the matrix, massive carbides in the matrix, and grain boundary at different ageing periods were evaluated, as shown in Figures 7A–C, respectively. With the increase in ageing periods, the Cr and Mo content in the massive matrix carbides increases and then decreases at longer ageing periods, while the Mo content in the grain boundary carbides sharply increases with the depletion of Cr and Mo from the matrix. The Mo depletion was found to be very faster compared to the Cr at high temperature exposure. The growth of carbides to their massive form is showing Mo rich with Cr and Mo depletion from the matrix, as reported earlier (13). The grain boundary carbides do not show enrichment of Cr and Mo at 300 h of ageing, whereas the enrichment was found at an ageing time ≥500 h. The martensitic lath structure no longer persists for ageing time ≥700 h, which was reported earlier with the simultaneous effect of high temperature and stress (8). The carbides were found to be in their massive forms at the longer ageing periods (≥700 h) with the coarsening of the finer carbides and found to be rich in Cr and Mo. Due to the coarsening of the carbides, the number density of carbides decrease with an increase in inter-carbide distance due to the transformation of MX type carbides to M23C6 or M6 C type carbides, as reported earlier (9, 13). Depletion of Mo from the matrix resulted in decrease in strength of the steel as Mo provides the solid solution that strengthens the steel. The accumulation of carbides at the grain boundary and their enrichment by Cr and Mo lead to the depletion of the elements adjacent to the grain boundaries, resulting in creep cavitation under high temperature and stress. With the coalescence of the cavities, cracks formed, and growth of the cracks led to failure of the structures in long-term service. Although such changes are occurring with cumulative change in microstructures in long-term service, it appears to be accidental one leading to the loss of material, machine downtime, and in some case manpower. Hence, monitoring the changes in the microstructure at regular intervals could reveal the health conditions of the components, and preventive measures could abort catastrophic failures in the power plants.

Figure 5. Microstructure of the martensitic 2.25Cr-lMo steel aged at 700 C for 300, 500, 700, and 1000 h, respectively, at 500X.

Figure 6. SEN Micrograph of the martensitic 2.25CrlMo steel (A–D) aged at 700″C for 300, 500, 700, and 1000 h, respectively, at 1000X, (E–H) are their corresponding micrographs at 5000X.
Microstructure of the steel at air-quenched condition is shown in Figures 8A–H aged at 700°C for 1, 3, 5, 25, 50, 75, and 100 h, respectively. SEM micrograph of the steel is shown in Figures 9A–D aged at 700°C for 1, 3, and 5 h, respectively, at low magnification (1000X), and (e)–(h) are their corresponding high-magnification micrographs (5000X). SEM micrograph of the steel aged at 700°C for 25, 50, 75, and 100 h is shown in Figures 10A–D at low magnification (1000X), and (e)–(h) are their corresponding high-magnification micrographs (5000X).

Figure 8. Microstructure of Bainitic 2-25Cr-lMo steel (A) as-air quenched condition (B–H) accelerated aged at 700 C for 1 h, 3 h, 5 h, 25 h, 50 h, 75 h and 100 h, respectively, at 500X magnification.

Figure 9. SEM micrograph Bainitic 2.25Cr-1Mo steel (A) as-air quenched condition (B–D) accelerated aged at 700 C for 1 h, 3 h, 5 h, respectively, (E–H) are their corresponding high magnification micrographs.

Figure 10. SENI micrograph of Bainitic 2.25Cr-lMo steel (A–D) accelerated aged at 700 C for 25 h, 50 h, 75 h, and 100 h, respectively, (E–H) are their corresponding high magnification micrographs.
3.1.2. Bainitic 2.25Cr-1Mo steel
Microstructure of the steel aged at 700°C for 300, 500, 700, and 1000 h at 500X is shown in Figures 11A–D. The corresponding SEM micrograph of the steel aged for different lengths of time from 300 to 1000 h is shown in Figures 12A–D at 1000X with their high-magnification micrograph at 5000X in Figures 12E–H. The EDX analyses of the massive carbides, matrix, and grain boundary carbides at different intervals were summarized, as shown in Figures 13A–C, respectively. A similar change was found with increase in Cr and Mo in the matrix massive carbides and their subsequent decrease at the longer ageing periods with a rapid increase in Mo at the grain boundaries, as mentioned in the martensitic steel. The depletion of Cr and Mo was found in the matrix, with the Mo depletion being pre-dominantly higher.

Figure 11. Microstructure of Bainitic 2.25Cr-lMo steel (A,D) accelerated aged at 700 C for 300 h, 500 h, 700 h, and 1000 h, respectively, at 500X magnification.

Figure 12. SEM micrograph of Bainitic 2.25Cr-lMo steel (A—D) accelerated aged at 700 C for 300 h, 500 h. 700 h, and 1000 h, respectively, (E–H) are their corresponding high.
3.2. Hardness
Microhardness of the martensitic and bainitic steel with ageing time is shown in Figure 14. Due to the presence of high density of dislocations and compressive residual stress along with the more martensitic lath boundaries, the hardness of the martensitic steel was found to be high at the as-quenched condition. With the thermal exposure at 700°C for 1 h, the annihilation of dislocations and relaxation of compressive residual stress resulted in a sharp decrease in the hardness of the martensitic steel, which is the effect of tempering the steel. Such change is quite less in the bainitic steel due to the presence of relatively less compressive residual stress and a relatively stable structure. With further increase in ageing time, the hardness decreases slowly due to the reduction in dislocations, residual stress, and martensitic lath broadening in the martensitic steel. The carbides formed and coarsened at long-term high-temperature exposure, leading to a decrease in number density and an increase in inter-carbide distance, which helped the easy movement of dislocations during indentation and decrease the hardness of the steel. Cr and Mo depletions are found in the matrix with their enrichment at the grain boundary carbides and in the massive coarsened carbides. The coarsening of carbides and the depletion of Cr and Mo from the matrix led to a decrease in the mechanical properties of the steel due to the softening of the matrix (1, 3). After 300 h of ageing, the martensitic steel showed poor strength compared to the bainitic steel due to the rapid deterioration of the metastable microstructure at high-temperature exposure.
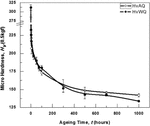
Figure 14. Change in micro hardness of the martensitic (WQ) andbainitic (AQ) 2.25Cr-lMo steel with increase in ageing time.
3.3. Magnetic properties
Magnetic hysteresis loop (MHL) is the B-H loop formed on application of a cyclic magnetic field to steel and recording magnetic induction (flux density per unit area). Coercivity or coercive force is the magnetizing force required to bring the residual magnetic field to zero. Magnetic domain wall obstruction caused by grain boundary/lath boundary, precipitations, and secondary phase results in higher residual magnetism remaining in a material after removal of the applied field. Hence, a large magnetizing force is required to remove the residual field, resulting in a higher coercive force. A decrease in pinning density in terms of microstructural degradation such as grain growth (which reduces grain boundary area), lath broadening (which reduces lath boundary area), spheroidization (which reduces the surface area of the carbides), coarsening of precipitates in the expenses of a large number of finer precipitates (which decreases the number density of precipitates), and relaxation of compressive residual stress (which reduces the strain field) decreases the coercive force in ferromagnetic materials such as steels (10–18).
The coercive force changes with time for the martensitic and bainitic steel, as shown in Figure 15. The coercive force is high at the as-quenched condition for the obstruction of magnetic domain wall by the martensitic lath boundaries, strain field of dislocations, and compressive residual stress. With thermal exposure to 1 h, the coercive force drops sharply which is similar to the change in microhardness, for the easy magnetic domain wall motion and the reduction of pinning density. However, the drop is much larger than the hardness due to the high sensitivity of the magnetic properties to the strain field of dislocations and residual stress. With further increases in ageing time, a slow decrease in coercive force was found, similar to the change in microhardness. The similar behavior of the coercive force and microhardness persists due to the common cause of change for both the magnetic domain wall motion and dislocation motion such as martensitic lath broadening, annihilation of dislocations, decrease in residual stress, increase in inter-carbide distance, disappearance of martensitic structure, and softening of matrix due to depletion of Cr and Mo. A decrease in coercive force was found for the martensitic structure after 300 h of ageing, and it is more prominent after 500 h of ageing due to the rapid deterioration of the metastable structure of martensite at high-temperature exposure.

Figure 15. Change in coercivity of the martensitic (WQ) and bainitic (AQ) 2.25Cr-lMo steel with increase in ageing time.
Plots of coercive force and hardness for martensitic and bainitic steel are shown in Figures 16, 17, respectively. The initial values of coercive force and hardness were not taken in the plots, as the material is used under tempered conditions, and hence 1 h of exposure is considered for the same. A strong correlation was found between the coercive force and hardness with a correlation coefficient of 0.96 and 0.97 due to the common mechanism of domain wall pinning and dislocation obstructions. Hence, by measuring the coercive force of the steel under service, the mechanical properties of the steel, which alter, due to microstructural degradation, could be monitored precisely using equation (1) for martensitic steel and equation (2) for bainitic steel.
4. Conclusion
Magnetic base NDE was employed to evaluate martensitic and bainitic 2.25Cr-1Mo steel after accelerated thermal ageing. Coercive force was correlated with the microhardness, which alter due to microstructural modifications. Grain boundary carbides with rich in Cr and Mo lead to the depletion of the elements at the matrix. Coarsening of such carbides to their massive form resulted in decrease in number density of carbides and increase in inter-carbide distance with a complete disappearance of martensite in the martensitic steel at the latter stage of ageing. Strong correlations were found between the coercive force and the microhardness of the steel, indicating precise evaluation of the mechanical properties of the steel in service by monitoring the coercive force. The study clearly shows that MHL could be a potential tool for the evaluation of microstructure and mechanical properties of Cr-Mo steels used in various power plant components by monitoring coercive force. Periodic monitoring of the components can help in assessing the health of the components for remedial/preventive action to abort catastrophic failure of the engineering components, whereas life extension of the components could be made, if the components are in healthy condition.
Author Contributions
JM had conducted the entire research work with the support of DK. Both authors contributed to the article and approved the submitted version.
Acknowledgments
We would like to acknowledge the support of JSW Steel Management for publishing the study.
Conflict of Interest
The authors declare that there is no monetary or personal conflict for carrying out the research and publishing the study.
References
1. Kim C, Park I. Microstructural degradation assessment in pressure vessel steel by harmonic generation technique. J Nucl Sci Technol. (2008) 45:1036–40.
2. Choi DH, Ahn BW, Yeon YM, Jung SB. Microstructures and mechanical properties of friction stir welded 2.25Cr1Mo Steel. Mater Trans. (2012) 53:1022–5.
3. Jha BB, Mishra BK, Satpati B, Ojha SN. Effect of thermal ageing on the evolution of microstructure and degradation of hardness of 2.25Cr-1Mo steel. Mater Sci (2010) 28:335–46.
4. Kumslytis V, Skindaras R, Valiulis AV. The structure and properties of 5% Cr-0.5% Mo steel welded joints after natural ageing and post-weld heat treatment. Mater Sci. (2012) 18:119–22.
5. Kim SH, Ryu WS, Kuk IH. Microstructure and mechanical properties of Cr-Mo steels for Nuclear Industries Applications. J Korean Nucl Soc. (1999) 31:561–71.
6. Mahajan Y, Peshwe DR. Failure analysis of 9%Cr-Mo steel component. Int J Eng Res Applic. (2014) 4:44–7.
7. Dobrzański J, Zielinski A, Krzton H. Mechanical properties and structureof the Cr-Mo-V low-alloyed steel after long-term service in creep condition. J Achiev Mater Manufact Eng. (2007) 23:39–42.
8. Kushima H, Watanabe T, Murata M, Kamihira K, Tanaka H, Kimura K. Metallographic Atlas for 2.25Cr-1Mo steel and degradation due to long-term service at elevated temperature. OMMI. (2007) 4:1–13.
9. Das SK, Joarder A, Mitra A. Magnetic Barkhausen emissions and microstructural degradation study in 1.25 Cr–0.50 Mo steel during high temperature exposure. NDT E Int. (2004) 37:243–51.
10. Kobayashi S, Tanaka M, Kimura T, Kamada Y. Changes of magnetic minor hysteresis loops, during creep in Cr-Mo-V Ferritic Steel. J Elect Eng. (2008) 59:29–32.
11. Won S, Hyun Y, Cho K, Lee J. Metallurgical failure characterization of 2.25Cr-1Mo Steel by magnetic property analysis. Key Eng Mater. (2004) 2004:70–4.
12. Byeon JW, Kwun SI, Hong SJ, Lee CK, Kang KM, Sohn YH. Correlation of magnetic barkhausen emission profile with strength of thermally degraded 2.25Chromium–1Molybdenum Steel. Mater Trans. (2005) 46:3089–91.
13. Mohapatra JN, Panda AK, Gunjan MK, Ratan Bandyopadhyay N. Ageing Behavior Study of 5Cr-0.5Mo steel by magnetic barkhausen emission and magnetic hysteresis loop technique. NDT E Int. (2007) 40:173–8.
14. Mohapatra JN, Panda AK, Mitra A. Magnetic properties evaluation of ageing behaviour in water-quenched 5Cr–0.5Mo steel. J Phys D Appl Phys. (2009) 42:095006.
15. Mohapatra JN, Bandyopadhyay NR, Gunjan MK, Mitra A. Study of high-temperature ageing and creep on bainitic 5Cr–0.5Mo steel by magnetic NDE techniques. J Magn Magn Mater. (2010) 322:589–95.
16. Mohapatra JN, Ray AK, Swaminathan J, Mitra A. Creep behaviour study of virgin and service exposed 5Cr–0.5Mo steel using magnetic Barkhausen emissions technique. J Magn Magn Mater. (2008) 320: 2284–90.
17. Mitra A, Mohapatra JN, Swaminathan J, Ghosh M. Magnetic evaluation of creep in modified 9Cr–1Mo steel. Scripta Mater. (2007) 57:813–6.